WARNING – This is a very technical article for Engineers. Enjoy. –Tiger
Topology Optimization in Medical Implants: Where are we now? (Engineering.com)
Last month we took a look at generative design in consumer products. We spoke to experts from CAD companies and manufacturers, and we learned that while there are instances of generative design in day-to-day products, they are still a way off from true mainstream adoption.
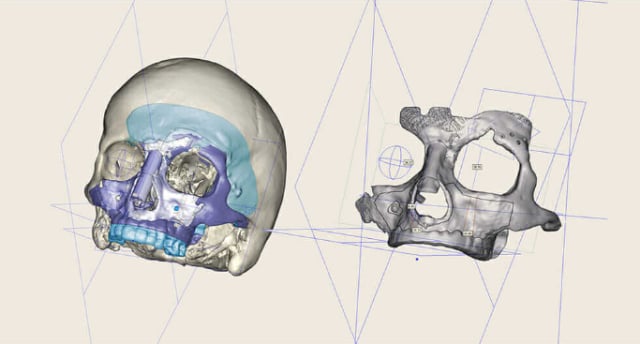
We learned that the biggest customers for generative design software are the manufacturers that require high-value, small production run, highly customized parts, such as aerospace companies and those involved in the medical implant industry.
So, to follow on the theme of last month’s article, we decided to speak to industry experts about the current state of generative design in the medical implant sector.
Implants
Medical implants, such as bone replacements, are the very definition of products that require a high level of customization. Every person is distinctly different, and so there is no one-size-fits-all solution where implants are concerned.
Therefore, it’s no surprise that biomechanical engineering companies have been early adopters of 3D printing technology. In fact, just recently the first recipient of a 3D printed titanium hip bone celebrated the 10th anniversary of the procedure—and the patient is reportedly still doing very well indeed. The surgeon who performed the procedure, Dr. Guido Grappiolo, has personally implanted over 600 3D-printed implants since that first procedure. Globally, over 10,000 patients have benefitted from implanted 3D-printed hip bone implants. That is a strong testament to the technology’s effectiveness.
But a lot has changed in those 10 years in terms of CAD and CAE simulation. Nowadays we have generative design and topology optimization, which can not only reduce the weight of these implants, but can also more closely mimic the trabecular structures found within bone.
So let’s once more ask the same question that we have been asking in this series of articles:
Generative design and topology optimization in medical implants: where are we now?
Altair/OptiStruct
It would be negligent to begin an article on medical implants and topology optimization/generative design without first discussing OptiStruct.
OptiStruct, which was the first commercially available topology optimization program, was born out of research into bone growth at University of Michigan. Altair’s chief marketing officer, Jeff Brennan, was investigating how bone growth responds to external stimuli, and was working on developing a mathematical algorithm to mimic this phenomenon (this theory is known as Wolff’s Law). Brennan later went on to work at Altair, and he took his research with him.
After a period of collaboration between the university and Altair, the company assumed responsibility for the development of the algorithm, and in 1994 released it as part of the OptiStruct platform.
Since then, OptiStruct has become one of the leading platforms for topology optimization and has branched out into a number of industrial applications, including aerospace and automotive.
And now, thanks to the magic of additive manufacturing, OptiStruct is returning to its roots and is being used to design bone implants. The product has come full circle. Effectively, the history of topology optimization (and by extension, generative design) is rooted in mimicking the natural behavior of bone growth in the natural world. Mother Nature is one heck of a design engineer.
The OptiStruct solver is available within Altair HyperWorks, as well as within solidThinking Inspire.
“OptiStruct is the structural solver and optimizer that provides topology optimization technology, but this tool is designed for a simulation specialist to use,” said Yuhao He, senior project engineer at Altair. “solidThinking Inspire packages OptiStruct’s capability with a more user-friendly interface to enable design engineers to conduct topology optimization/ generative design in early stage.”
The OptiStruct solver in its various forms has been used to design a hip implant, a jaw implant and a spine insert.
But, aside from the high degree of customization that 3D printing allows, and the weight reduction offered by topology optimization, what other advantages are there to designing implants with generative design?
“For the hip implant that we designed, the advantage is that it can reduce stress shielding and bone resorption by its optimized stiffness,” said He.“Bone resorptionis one of the major causes of implant loosening and high bone fracture possibility.” Bone resorption is the loss of bone material caused by the osteoclasts breaking down the bone tissue.
This is a huge concern, as implant loosening and dislocation are the two leading causes of revision surgery in total hip arthroplasty (THA). Simply put, a well-designed implant will last longer in the patient, and require less corrective surgery down the road.
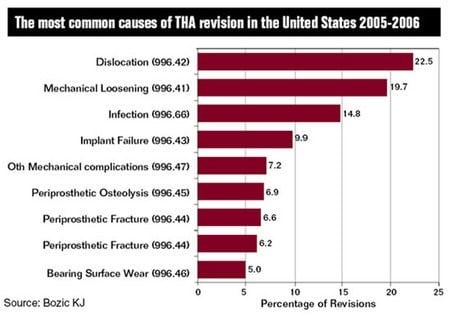
Stress shielding is an important concept, and one that we will return to later, so it is worth clarifying the term here.
Titanium is a preferred material in implants because it is biocompatible. But the problem is that titanium is 6.5 times stiffer than the cortical bone in the human femur. As a result, when a titanium implant is placed inside a patient, the load distribution into the natural bone changes (it is reduced) causing stress shielding. And as per Wolff’s Law, the bone remodels itself based on the loads that it experiences. As there is a reduced load with a titanium implant, the bone actually loses mass, and or bone resorption can occur, and that’s when fractures, dislocation and loosening can happen.
So, designing an implant that is as close to the original material as possible on the micro-structural level is the key to distributing these loads naturally—and is therefore the key to reducing these unwanted effects.
Figure 3 shows various von Mises stress plots of a femur. The first image shows the natural, healthy bone. The second image shows the femur with a traditional implant, and the third image shows the stresses on the femur with the optimized implant. Note that the third image with the optimized implant shows a similar stress concentration to the original healthy bone. This is desirable. The second image shows reduced stress. This demonstrates stress shielding, which is not desired.
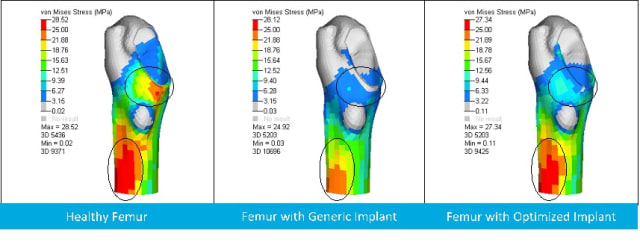
Altair and solidThinking use OptiStruct to design optimized lattices that reduce stiffness and mass, and therefore reducing stress shielding.
“The algorithm is robust and allows manufacturing limitation like overhang angle for 3D printing to be considered,” continued He. “Recently, it enabled users to create lattice or semi-lattice structures [in which the]lattice distribution and diameter of each ligament are all optimized.” You can see the lattices in Figure 4.
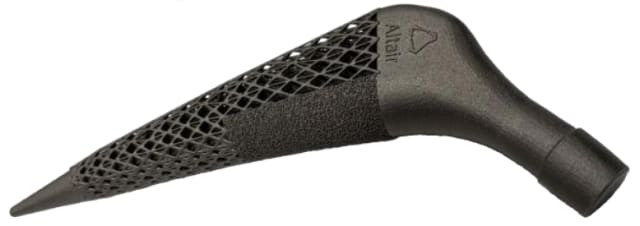
“Combined with additive manufacturing, topology optimization enables engineers to design customized implants based on patients’ anatomy, activity level and bone mechanical properties. Those customized implants will be more compatible with the specific patient and still sufficiently strong.”
Frustum Inc.
Frustum is a relative newcomer to the CAD realm, having been founded in 2014 by former Columbia University professor Jesse Coors-Blankenship.
Frustum offers software named Generate, which is a cloud-based generative design/topology optimization solution that focuses on mesostructures. It has also been used to design medical implants such as hip stems, hip sockets and knee implants.
We spoke to the founder and CEO to see how its solution differs from others on the market.
“While other generative design software helps arrive at an end result through a sequence of operations, Generate is interactive, so any parameter changed immediately updates the generative design,” said Jesse Coors-Blankenship. “This makes generative design functional, as Generate‘s output geometry does not have to be reworked or finessed in post-processing like others. This means huge time savings and higher quality parts.”
You can see a hipbone cup that was designed in Generate in Figure 5.
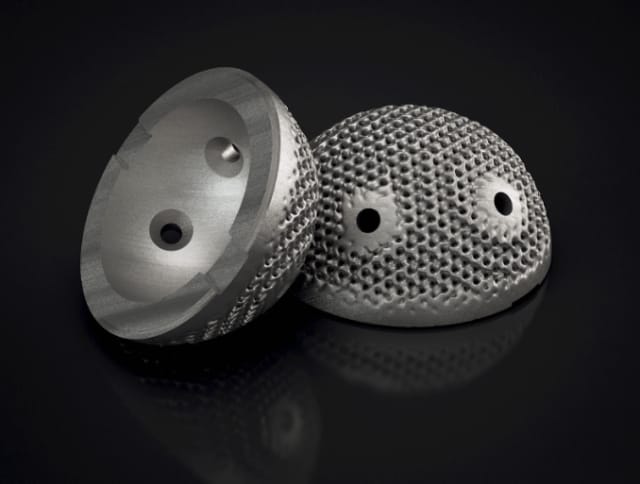
So, what are the advantages of these optimized/generatively designed implants compared to those that have been traditionally manufactured?
“Primarily, more sensitive customization to the patient and ideal lattice structures that promote healthy bone growth that minimizes decay,” said Coors-Blakenship. The decay he is referring to is the loss of bone mass caused by resorption, as we discussed earlier.
We have discussed Frustum and its software in previous articles, and you can read more about the company’s Generate software at this link.
ANSYS
ANSYS is another big player in generative design and topology optimization, and so naturally it has been heavily involved in medical implant design, working primarily on spinal cages and other orthopaedic implants.
The ANSYS platform (currently on ANSYS 19.1) features a vast array of tools for simulation and modeling, but the topology optimization feature can be found in the ANSYS Mechanical product. ANSYS topology optimization has been used extensively in aerospace, automotive, energy and consumer products, according to Steve Pilz, lead product manager at ANSYS.
Customers are aware of the benefits of topology optimized implants.
“Benefits vary from optimized implants being lighter weight to having a longer useful life via improved robustness.” said Pilz. “Additional benefits range from lower final manufactured cost to reduced rejection rates to superior biocompatibility via custom patient fitting.”
What distinguishes the ANSYS products from others in this market is the interconnectability across the entire ANSYS product range.
“With ANSYS Topology Optimization capabilities being built into Workbench, all other Workbench applications work seamlessly with it. Functionality such as geometry smoothing, support structure generation, additive print process simulation and extensive validation capabilities are simple and repeatable,” explained Pilz.
Although the focus of this article has been largely on bone replacement implants, ANSYS has been involved in a number of other implant types and has worked with a number of companies on a variety of products.
These include Medtronic (neurostimulation and orthopaedic), Stryker (orthopaedic), Johnson &Johnson (orthopaedic), and Cyberonics (neurostimulation).
Autodesk
Porosity is a big thing when it comes to creating implants that are designed to replace bone. The direction of the material between the pores guides the forces acting on the bone correctly and reduces stress shielding, as we discussed earlier.
Autodesk has a product that initially had its roots in generative design named Autodesk Within Medical. This product is tailored toward medical implant design and allows users to vary the lattices within the implant models to allow for greater osseointegration.
Osseointegration refers to the integration of bone and implant, and aside from the load-diverting properties of porous microstructures in bone, is a key design driver for the inclusion of pores in implant design.
The mechanism by which implant and bone bonding occurs is the same as how a bone fracture heals itself. The presence of pores promotes better growth at the interface between the bone and implant, and provides a better geometric anchor once osteogenesis begins (like a jigsaw puzzle; see Figure 6).
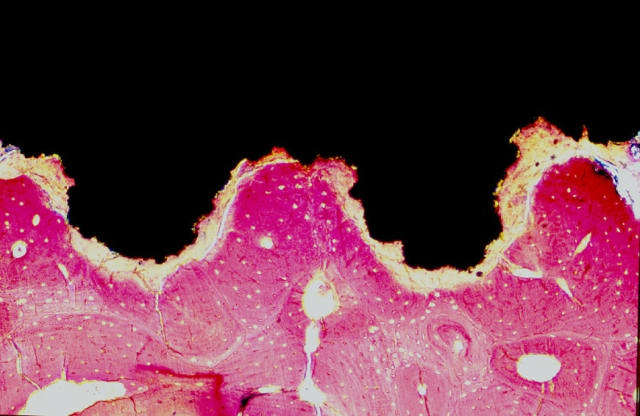
These principles of pore optimization have guided the development of Autodesk Within Medical. And now, thanks to this software, surgeons, bioengineers and prosthetists have a new tool to help design implants that provide better compatibility, comfort and safety.
Take, for example, the case of the titanium facial implant, as shown in Figure 7. This implant was designed by Slovakian company CEIT Biomedical Engineering for use in a patient who had sustained severe facial injuries in a car accident.
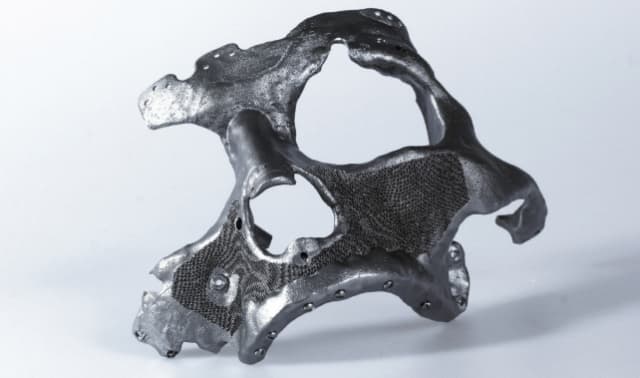
A decade ago, such an implant would have been impossible to design optimally, and impossible to manufacture with traditional methods.
“The use of subtractive methods using CNC machines was very complicated,” said CEIT Biomedical Engineering co-owner Radovan Hudak. “It is impossible to make the thin walls needed to create porous structures in different shapes using traditional milling machines.”
The porous nature of the facial implant allows osteoblasts to permeate the structure, and promotes growth of fresh bone at the interface between the bone and the implant, thus allowing greater osseointegration.
“The world is changing quickly with new materials, techniques and machines being developed all the time,” Hudak said. “This is a truly exciting time for medical implant development and innovation.”
The face implant was so successful that it allowed an 86 percent restoration of maxillofacial bone tissue, making it the most extensive maxillofacial implant manufactured by additive manufacturing and in the history of the industry. And because it was designed in Autodesk Within Medical, it is also the most extensive facial implant that was designed in a topology optimization-derived software platform!
So that is good news. We can see that topology optimization and generative design are making a real difference to peoples’ lives, and the technology isn’t just being used to create fancy graphics for marketing.
Paramatters
Last, but by no means least, we spoke to U.S.-based Paramatters, which, like Frustum, is a new company, although its founder, Michael Bogomolny, has plenty of generative design experience under his belt, having previously worked in software development on OptiStruct.
Paramatters released its cloud-based generative design platform, CogniCAD, earlier this year, and is promising one particular feature that not even Altair has offered yet. Its CogniCAD platform offers optimal porosity in structures that are able to emulate the microstructure of bone more accurately.
In the other software packages, the generative designed bone porosity tends to appear as regular, repeating patterns (or lattices) as you have seen. This, according to Bogomolny, is not how bone grows in real life. When you look at bone microstructure under a microscope, you can see that the porosity and structure aligns itself in the direction of force, resulting in “grains” or trabecular structures that vary in direction and size, depending on how the load runs through the bone. This is illustrated in the image of a femur bone shown in Figure 8.
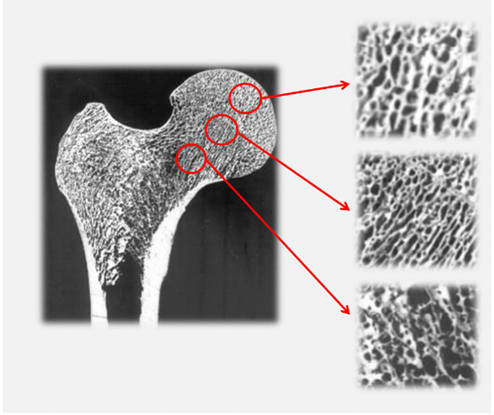
The algorithm in CogniCAD replicates this varying porosity, providing an optimized bone structure that is lightweight, allows better compatibility with natural bone, and can even allow the bone to flex, just like a real bone. The increased flex and optimized load paths prevent stress shielding as mentioned earlier, and make for more comfortable, long-lasting implants.
“We want to see a one-click solution. With Altair and these other tools, we really see topology optimization as a hint of how a design should look. And once the optimized design is done, you have to go back and reconstruct the geometry manually. Our technology eliminates all of this process. You design your problem, as you usually do in topology optimization, in terms of design domain, boundary conditions and design goals, and with our one-click solution, you get this watertight design that is ready for printing.
“You don’t want to replicate the bone as lattice everywhere.
You want the right material distribution….We developed this piece of technology that we call the mesostructural topology. During the generative design process, it [the simulation] converges to microstructures that align with the principal stresses. If I were to run a regular topology optimization on this case, we would get just bars…regular bar-like, lattice structures. But this technology allows us to generate optimal porosity. By the way, we don’t call our structures ‘lattices’…we call them ’metamaterials.’
“To give you an idea, to get a design that looks like this, we needed to go to a resolution of 30 million elements…that’s like 90 million degrees of freedom—it’s a huge problem. Imagine, to generate this design, we need to resolve this complicated set of equations through about 70 iterations until the topology optimization converges. But numerically, our technology is there. This is unique in what we do. We are bone mimicking where the design solution aligns with the principle stresses. And we believe that bone mimicking has a lot of potential,” said Bogomolny.
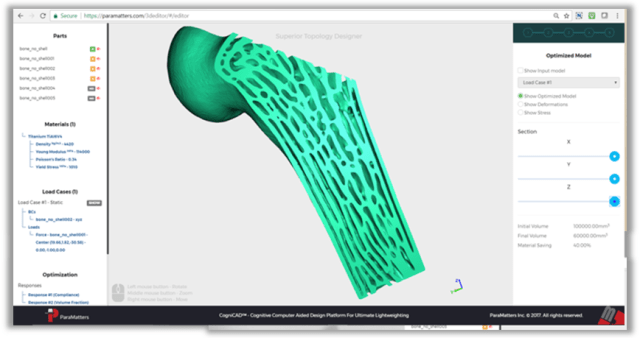
“What we have learned is that if an implant is too stiff, then the remaining bone has to work less and eventually just disappears.
“What you would ideally like to have in an implant is an implant that is itself as close as possible to the real bone properties. Obviously, clinical trials need to be conducted, but it’s a good engineering guess to say that if the implant properties are the same as the bone properties, then this will be good from all perspectives. What our technology allows is for us to tailor the implant properties as close as possible to that of bone. I’m not aware of any other technology on the market that can do this,” explained Bogomolny.
CogniCAD is available now, and the porosity optimization solution (mesostructural topology optimization) is available as a fully functioning code for design projects. You can contact Paramatters for more details.
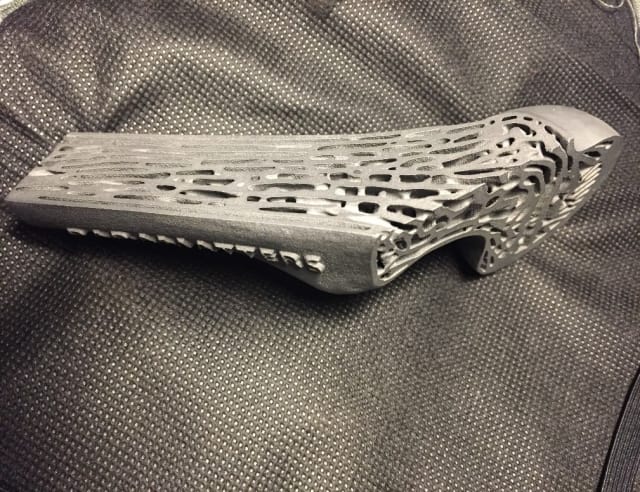
Final Thoughts
One thing about these bone implants that you may have noticed is that the overall shape of the implant has remained the same as the part that it was designed to replace.
With topology optimization in consumer goods or even in high-end engineering goods, the trend in topology optimization/generative design tends to show that the large-scale shape of the structure is changed in the optimized product.
What we see here in medical implants, and in particular in bone replacements, is that the large-scale shape remains the same, and that most of the optimization occurs at the microstructural level.
While there is space to be creative in design engineering, when it comes to implant design, high fidelity is king. Faithful reproductions of the structure at the macro level mean that patients can get on with their lives, walk normally, and feel normal, while true-to-life bone emulation at the micro level enables better osseointegration, and allows for loads to be diverted in the correct fashion.
Sometimes the wackiness that we come to expect from generative design isn’t quite yet wacky enough to match Mother Nature.